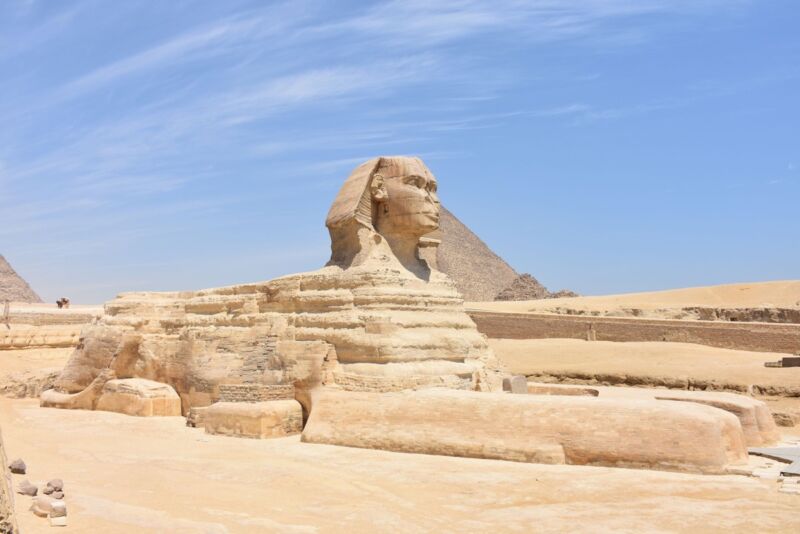
Leif Ristroph, a physicist and applied mathematician at New York University, was conducting experiments on how clay erodes in response to flowing water when he noticed tiny shapes emerging that resembled seated lions—in essence, miniature versions of the Great Sphinx of Giza in Egypt. Further experiments provided evidence in support of a longstanding hypothesis that natural processes first created a land formation known as a yardang, after which humans added additional details to create the final statue. Initial results were first presented last year as part of the American Physical Society's Gallery of Fluid Motion, with a full paper being published this week in the journal Physical Review Fluids.
"Our results suggest that Sphinx-like structures can form under fairly commonplace conditions," Ristroph et al. wrote in their paper. "These findings hardly resolve the mysteries behind yardangs and the Great Sphinx, but perhaps they provoke us to wonder what awe-inspiring landforms ancient people could have encountered in the deserts of Egypt and why they might have envisioned a fantastic creature."
In 2018, Ristroph's applied mathematics lab fine-tuned the recipe for blowing the perfect bubble based on experiments with soapy thin films, pinpointing exactly what wind speed is needed to push out the film and cause it to form a bubble, and how that speed depends on parameters like the size of the wand. (You want a circular wand with a 1.5-inch perimeter, and you should gently blow at a consistent 6.9 cm/s.)
Last year, Ristroph's group conducted a series of experiments involving paper airplanes to explore the underlying aerodynamics, developing a handy mathematical model to predict flight stability. It was already well-known that displacing the center of mass results in various flight trajectories, some more stable than others.
The team verified this by test-flying various rectangular sheets of paper, changing the front weight by adding thin metallic tape to one edge. If the weight was centered, or nearly so, at the center of the wing, the plate would flutter and tumble erratically. Displace the center of mass too far toward one edge, and the plate would rapidly nosedive and crash. The proverbial "sweet spot" was placing the weight between those extremes. In that case, the aerodynamic force on the plane's wing will push the wing back down if it moves upward and push the wing back up if it moves downward. In other words, the center of pressure will vary with the flight angle, thereby ensuring stability.
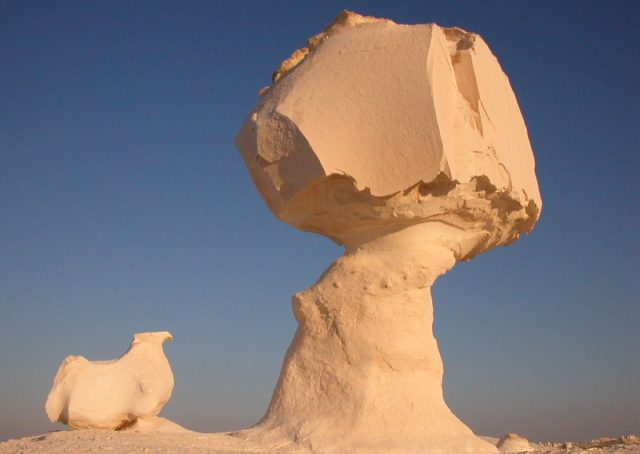
Most relevant to this latest study is Ristroph's 2020 paper on so-called "stone forests" common in certain regions of China and Madagascar (technically a type of karst topography), like the famed Stone Forest in China's Yunnan Province. They conducted simulations and experiments to explore the interesting shapes that evolve in landscapes due to a number of "shaping" processes, most notably erosion and dissolving.
Ristroph et al. concluded that these pointed rock formations result from solids dissolving into liquids in the presence of gravity, which produces natural convective flows. Soluble rocks like limestone, dolomite, and gypsum are submerged under water, where the minerals slowly dissolve into the surrounding water. The heavier water then sinks under the downward pull of gravity, and the flows gradually form karst topographies. When the water recedes, the pillars and stone forests emerge.
reader comments
33